Giant excitons in interaction
Recent articles in Nature Communications co-authored by prof. Thomas Pohl, demonstrates how excited quantum states can be used to control and exploit strong interactions in semiconductors.
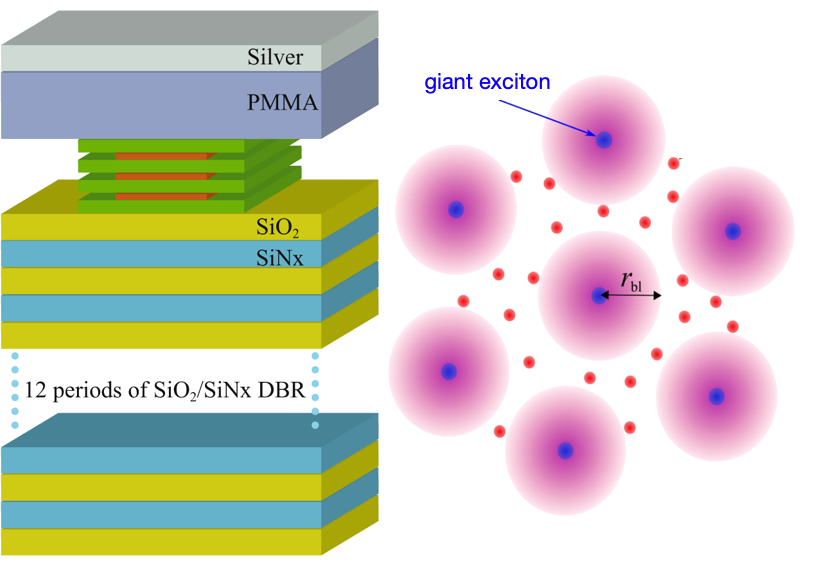
Giant excitons in interaction
Semiconductor excitons have become indispensable for modern optical technologies. Two international research teams, including Thomas Pohl from the Center for Complex Quantum Systems, now demonstrate how excited quantum states can be used to control and exploit strong interactions between such excitons. The results have been reported in two recent articles in Nature Communications.
When light interacts with a semiconductor, it can liberate electrons from their atomic cores, which leaves behind a positively charged hole. The two charges, created in this way, can freely move through the material and bind together by their mutual Coulomb attraction. Such electron-hole bound states — or excitons — provide a solid state analogue of simple atoms and are at the heart of modern optics and laser technology. Due to their relatively small size, however, excitons only interact very weakly, which limits the extent to which nonlinear optical processes could be studied so far.
New research from two international collaborations, including the Center for Complex Quantum Systems, now shows that excited states of excitons can be used to generate and control strong interactions between excitons. The bound electron-hole state behaves very much like a Bohr atom and as such also exhibits excited bound states. A recent work from the Technical University of Dortmund, the University of Rostock and Aarhus University succeeded to simultaneously create excitons in two different excited states by shining photons with two different frequencies onto a cuprous oxide semiconductor. The used material has been of exceptional quality, which made it possible to create excitons in very highly excited states with remarkable sizes in the micrometer domain. Such extreme excitons have record-breaking interactions which exceed that of regular excitons by many orders of magnitudes. The reported work succeeded to directly probe such gigantic interactions for the first time in experiments and represents a key advance towards realizing highly nonlinear optical processes.
Another important step towards this goal has been made in a recent collaboration between the City College of New York, Stanford University, Columbia University, Lawrence Berkeley National Laboratory, Polytechnique Montréal and Aarhus University. Here, the researchers succeeded to realize strong coupling between photons and excitons by embedding a two-dimensional semiconductor inside an optical resonator, formed by planar microscale Bragg mirrors. Under such conditions the resonator photons start to hybridize with the excitons in the two-dimensional quantum material and form a quasiparticle, termed polariton. The reported work made it possible to form such cavity polaritons with excited-state excitons for the first time in experiment, and demonstrated that this gives indeed rise to an enhanced optical nonlinearity, corresponding to an enhanced effective interaction between photons inside the optical cavity. While the size of the excited exciton could not yet reach the dimensions possible in cuprous oxide, as described above, the observed enhancement in an optical resonator present a vital and encouraging step.
Generally, the ability to enhance photon coupling in optical resonators and to obtain strong exciton interactions in excited states, yields an ideal combination for exploring nonlinear optical processes. Such explorations are now possible in two rather different and complementary materials and give a promising outlook on optical science and quantum technologies with individual photons.
The described research has been recently published here and here.